Prediction of fluid responsiveness in ventilated critically ill patients
Introduction
Circulatory shock is a life-threatening condition, it is defined as a poor oxygen utilization by cells due to an acute circulatory failure, it can be distinct in various subgroups: hypovolemic, distributive, cardiogenic and obstructive (1). Reducing tissue hypo-perfusion is the most important purpose to pursue in this critically ill patients, this goal can be obtained with administration of intravenous fluids, correcting hypovolemia increasing venous return, cardiac preload and thus cardiac output (2) In patient with septic shock, the 2018 updated guidelines recommend to start fluid resuscitation with a minimum of 30 mm pro kilo of crystalloids, to be completed no later than 3 hours after diagnosis (3). However, the initial infusion is not always enough to correct tissue hypoxia and neither to control cardiovascular instability, at this point the decision to continue fluid administration can be challenging for the Intensive Care Physician. Most studies have showed that just part of severely ill patients benefit from this therapy (4), such patients present an increase of cardiac output after a fluid administration and are called “fluid responder” (5), the most common definition of fluid responsiveness being an increase of stroke volume of 10–15% after administration of 500 mL of crystalloids in 10–15 minutes (6). On the other hand, patients that don’t respond to fluid administration are more likely to develop a condition of fluid overload (7). Fluid overload can result in many adverse effects, without any hemodynamic benefits, like: tissue edema, delays in weaning from mechanical ventilation, increased length in ICU and hospital stays and it is also a predictor of increased mortality in septic shock, acute distress respiratory syndrome (ARDS), intra-abdominal hypertension and acute kidney injury (1,6-8). This data underlines the importance of identify sign of fluid responsiveness in critically ill patients, in other words those who are capable to respond to an increase in circulating volume with an improvement in cardiac performance.
Static measurements
Measurements of fluid responsiveness can be static or dynamic. Static measures have been used for the last decades and are used to estimate preload (venous return). Preload in steady-state conditions is equal to cardiac output. Mathematically, preload is determined by the relationship of venous resistance with systemic filling pressure and right atrial pressure (1); venous capacitance is important because influences venous return and central venous pressure (CVP) (2). CVP is the most important static measurement. Further static measures are pulmonary artery occlusion pressure (PAOP), right atrial pressure (RAP) left-ventricular end-diastolic area (LVEDA), inferior vena cava diameter (IVC), right ventricular end-diastolic volume (RVEDV).
Central venous pressure
Multiple modern studies showed CVP/ΔCVP as imprecise and unreliable to predict fluid responsiveness because of a weak relationship between CVP and blood volume; CVP monitoring requires a catheter placed in superior vena cava near the right atrium junction; furthermore, the evaluation of CVP has not a standard pattern although frequently is determined immediately after the atrial contraction (A wave) and the valve closure (C wave) (3). The CVP correspond to right atrial pressure (RAP). Right ventricular output is commensurate to left-ventricular filling but may not reflect the actual filling pressure (4,5): a low RAP indicate the ascending limb of the Frank-Starling curve; a high RAP suggest that the patient is on the plateau (1), although it varies from one patient to someone else, and in the same patient, between different times (6). Static pressures and fluid responsiveness have a few relationship because of variations in venous tone, intrathoracic pressures, ventricular functions. This determine the few link between CVP and RVEDV. Thusly, a ventricular preload could be associated to the existence of ventricular preload reserve even with healthy heart-function or in absence of ventricular preload reserve (decreased contractility). Although CVP has a low accuracy for predicting fluid responsiveness, it is widely used with other static markers to test preload responsiveness in 1/3 of cases, as shown by the FENICE study (7), an observational study operated in intensive care units all over the Earth. In a study about hemodynamic monitoring in patients under high-risk surgery, 73% of American and 84% of European anesthesiologists reported the use of CVP to govern fluid management (8). A systematic review including 803 patients analyzed CVP with measured circulating blood volume and the relationship between CVP/ΔCVP following a fluid challenge. The difference in CVP at baseline in responders (8.7±2.32 mmHg) versus non-responders (9.7±2.2 mmHg) resulted not statistically significant (P=0.3) (4).
Pulmonary artery occlusion pressure
Pulmonary artery catheters (PACs) measure the PAOP which corresponds to the end-diastolic pressure of the left-ventricular (LVEDP) (1). PAOP could be subject to several valuables: compliance of myocardial tissue alternated (as in septic state or ischemia), pericarditis, increase of pulmonary vascular resistance, right ventricular overload, mitral stenosis and increased intrathoracic pressure due to mechanical ventilation (9). Moreover, to insert a PAC is an invasive operation with risk of arrhythmias, pulmonary infarction, catheter knotting, and rupture of the vessels and its routine use is not recommended in critically ill patients (1). In a study with 96 patients, Osman et al. evaluated the relationship between the evaluation of PAOP and patients “fluid responders”: responders had smaller values of PAOP before the infusion than non-responders (10±4 vs. 11±4 mmHg, P=0.05), but they noted a big overlapping of values in different persons. The area under ROC curve misurated was not statistically significant compared to that misurated for CVP: 0.63 (95% CI: 0.55–0.70); the difference between the two under the ROC curves areas was 0.053 (95% CI: 0.01–0.12; P=0.12) (10). A study by Michard et al. measured PAOP before and after a fluid increase in the two categories of patients: responders and non-responders; they showed a not-significant evidence of measurement of PAOP in seven of nine studies (11).
Global end-diastolic volume (GEDV)
GEDV and the derived global end-diastolic volume index (GEDI) estimate the blood volume of all the four cardiac chambers with the technique of transpulmonary thermodilution (TPTD). PiCCO® (Pulse Contour Cardiac Output) monitor or EV1000 monitor (Vigileo®) are employed to obtain these measurements. The monitoring-system PiCCO utilizes TPTD method through a central venous catheter (CVC) and a thermodilution-tipped arterial catheter; it can measure multiple variables: GEDV/GEDI is an evaluation of preload and of stroke volume because estimates the volume in the four hearth-chambers, although the relationship between GEDV-measurement and patients fluid responders is lacking. Venous capacitance and heart chambers compliance are important to identify a change in preload, influenced by different GEDV values (11). Michard et al. reported a significant association with stroke volume index (SVI) (r=0.72, P=0.001) in 36 patients comparing GEDI with SVI before and after a fluid challenge; moreover, pre-infusion GEDI was smaller in patients fluid responders than in non-responders (637±134 vs. 781±161 mL/m2, P=0.001) (12). Endo et al. found GEDV to be unpredictable in prediction of fluid responsiveness on 93 mechanically ventilated patients (13). Due to the poor data and the heterogeneity of the results about GEDV/GEDI to predict fluid responsiveness, further research is needed.
Inferior vena cava diameter
The diameter of the inferior vena cava (IVC) theoretically changes in relation to preload and its increase should correspond to an increment in preload and right atrial filling pressure. The IVC can be measured through ultrasound at a determined length from the right atrial cavity, during the expiratory time (Figure 1). Its accuracy is operator-dependent and needs image acquiring and analysis. Many components like obesity, lung hyperinflation pneumothorax, abdominal distention or elevated intra-abdominal pressure (>12 mmHg) may cause unsatisfying sonographic windows. The diameter of the IVC is correlated with RAP (14,15). In a multicenter study, in 22% of patients was not possible to obtain the IVCd; in only 29% of the ICU-ventilated patients was possible to predict fluid responsiveness with a specificity of 80%. Even so, a value of end-expiratory IVCd less than 8 mm or more than 28 mm could predict patients fluid responders with a specificity of 95% (16).
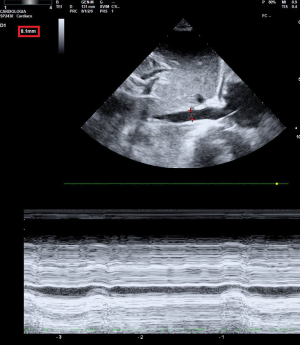
Left ventricular end-diastolic area (LVEDA)
LVEDA is estimate with transthoracic echocardiogram (apical 4-chamber view) or transesophageal echocardiography (TEE) (Figure 2) and should increase with fluid expansion in responding subjects. Feissel et al. considered LVEDA at end-expiration phase before and after fluid expansion in ICU ill patients with TEE. They found no convincing interaction (r2=0.11, P=0.17) between LVEDA index measured at a standard time and the increment in cardiac index in response to fluid expansion (17). Tavernier et al. compared different hemodynamic variables and found that LVEDA had a lower area under the ROC curve compared to systolic pressure variation (SPV) (0.77, 95% CI: 0.59–0.92 vs. 0.94, 95% CI: 0.81–0.99) (3,18-21). Michard et al. analyzed twelve studies and showed that static indicators of cardiac preload (RAP, PAOP, RVEDV, LVEDA) in patients in ICU, before fluid infusion, were not significantly decreased in responders than in non-responders (11). Not even the CVP (4,10), as well the PAOP (10,22), LVEDA (23,24), the early/late diastolic wave ratio (24), or the B-type natriuretic peptide blood-concentration (25) can contradistinguish responsiveness to fluid therapy in patients because there are many curves that characterize the correlation between stroke volume and ventricular preload, cause of the contractile function of ventricles; moreover a confounding factor is the transmission to the cardiac chambers of the pleural compression.
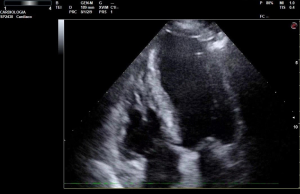
E/e’
LV diastolic dysfunction can be defined as an increase in myocardial stiffness or a reduction in the rate of relaxation of the heart muscle (26).
This condition can be assessed echocardiographically, with the tissue Doppler, through indices like e’ and E/e’; the e’ wave provides information on the maximum speed of movement of the mitral annulus during the rapid ventricular filling phase; the E wave represents the maximum velocity of the rapid ventricular filling; E/e’ is the ratio between these two values (27,28).
Values of e’ less than 7 cm·s−1 for septal and less than 10 cm·s−1 for lateral tissue velocity, are considered abnormal. The values of the ratio E/e’ correlate with the LAP (left atrial pressure) and with the capillary Wedge pressure, values below 8 indicate a non-elevated LAP, values above 14 indicate an increase in the filling pressures of the left heart chambers (29).
The alteration of the correct release of LV can lead critically ill patients to heart failure, pulmonary edema and difficulty in weaning from the mechanical ventilator (30). During circulatory failure, fluid administration is often used to increase stroke volume; if we assume that this volume of liquids, we infuse is able to correct (at least partially) the LVDD, the variables of e’ and E/e’ seems useful and reliable for testing this hypothesis (31-33).
In the study of Mahjoub et al. it has been shown that the administration of liquids (500 mL of saline solution) causes an increase in the values of e’ higher in the patients considered responders (SV increased more than 15%) on the other hand the value of E/e’ had increased more markedly in the group of non-responders (34,35).
E/e’ ratio proved to be a good predictive value of LV filling pressures in the patient with septic shock. (11). Sanfilippo et al found a significant correlation between mortality in critically ill patients and low levels of e’ and high values of E/e’ patients.
The assessment of LVDD in critically ill patients remains difficult and the evaluation of E and E / e is probably the most used tool because it is easy to perform at patients’ bedside (36).
Dynamic measures of preload responsiveness
The interactions between heart and lungs are the starting point for almost all tests and dynamic measurements that can be performed in patients subjected to mechanical ventilation. During the respiratory cycle many changes in pressure and load occur in the heart chambers (37). In the inspiratory phase, there is a decrease in preloading of the right atrium due to the transmission of intrathoracic pressure, which increases at this time in the cycle (1,38). Fluid responsiveness has a higher incidence in patients with more pronounced responses in decreasing preload. Patients with higher stroke volume modifications during a respiratory cycle in mechanical ventilation can be more easily preload responsive (37,39).
Stroke volume variation
The first method to be developed for the dynamic valuation of preload responsiveness is the stroke volume variation (SVV) (Figure 3). This parameter goes up during inhalation and goes down during exhalation due to changes in intra-thoracic pressure caused by positive pressure ventilation. The rationale can be that, during positive pressure ventilation, insufflation decreases preload of the right ventricle. When end positive pressure is transmitted to the left side, it causes a decrease in preload of the left ventricle (40). If left ventricular stroke volume changes in response to cyclic positive pressure ventilation, this indicates that both ventricles are preload dependent. A larger SVV variation suggests that the patient will be fluid responsive. Traditionally, the threshold for fluid responsiveness is SVV >13% (1,41-45). Zhang et al. showed in their meta-analysis that SVV can predict fluid responsiveness with a diagnostic odds ratio of 18.4 at a sensitivity of 0.81 and specificity of 0.80. One of the main limits of the study is that it has proved to be reliable only in mechanically ventilated patients (1,46). The SVV may not be completely reliable in patients who breathe spontaneously, especially those with respiratory distress, consider the irregularities in the variations of the intrathoracic pressures. Another limit to consider is the need to set the ventilation parameters with a tidal of at least 8–10 mL per kilo. The predictive power of SVV decreases with minor tidal settings (1,47,48) and also in patients with cardiac arrhythmias (1,42,44,49,50).
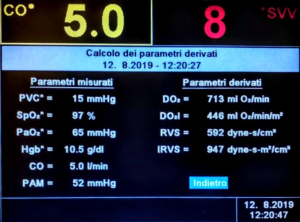
Pulse pressure variation (PPV)
The definition of this parameter is given by the differences in pulse pressure during the respiratory phases. We can obtain this parameter by placing a catheter in an arterial vessel and observing the variation of the waveform and calculating the blood pressure modifications during the phases of systole and diastole (1). Patients with septic shock and subjected to mechanical ventilation were studied by Michard et al., their work showed that PPV can predict fluid responsiveness with a threshold of 13% (37,51-53). There are some limitations, this parameter is not reliable in patients with open chest, in those who breathe spontaneously, low tidal volume or high-frequency ventilation, cardiac arrhythmias, intra-abdominal hypertension and in patients with low lung compliance (37,54,55). regarding the latter limitation the cut-of seems to be a compliance less than 30 mL/cmH2O, in this case the value of PPV in predicting fluid responsiveness is intensely decreased (1,55). These limitations come into play for intermediate values of PPV (greater than 9% and less than 13%) there are still few reliable data (1,56). A step forward was made by Yang et al. who recently proposed a meta-analysis that included patients with tidal volume of less than 8 ml per kilo with interesting results, PPV values have been shown to possess a sensitivity of 0.88 (95% CI: 0.81–0.92), a specificity of 0.89 (95% CI: 0.84–0.92), and AUROC of 0.94 (95% CI: 0.91–0.95) (1,53). Another work regarding this field is the one by Myatra et al., the patients considered had a very low tidal value (6 mL/kg), they put into effect a protocol in which the tidal was increased from 6 to 8 mL per kilo for one minute and the value of PPV was annotated, significant increases of 3.5% were considered (37,41).
Systolic pressure variation (SPV)
In both patients who breathe spontaneously and in those subjected to mechanical ventilation, there are physiological fluctuations in systolic blood pressure (1,50). In order to use this parameter, we must define a starting point of the pressure value, generally established after 7–12 seconds after an end expiratory pause. From this baseline the pressure can increase or decrease during the respiratory cycle, the delta Up (dUp) and delta-Down (dDown) values are thus recorded (1,18). The SVP is therefore defined as the difference between the highest and lowest values of the systolic pressure. Many studies observed that dDown can be a reliable predictor of fluid responsiveness (1,18,57). Tavernier et al. showed that a value of dDown >5 mmHg can have a positive prediction value of 95% and a negative prediction value of 93% (1,57). The limits of this method are that it is reliable only in mechanical ventilated patients without cardiac arrhythmias, on the other hand it is an inexpensive method (1).
Aortic velocity time integral (VTI)
This parameter is an echocardiographically obtained measure of the area under the velocimetric curve within the left ventricular outflow tract, or rather the distance travelled by the column of blood cells through the aforementioned tract during ventricular systole, which therefore allows to have a stroke volume measurement. The measurement is performed with transthoracic echocardiography, with 5-chamber apical projection and use of pulsed Doppler, normal values in adult patients at rest are between 18 and 22 cm, lower values are an index of reduced CO. In order to avoid interference related to the respiratory dynamics, it is preferred to carry out this measurement at the end of the exhalation, in this way also the patient’s preload status should not significantly interfere (58).
According to various studies in patients in sinus rhythm it is sufficient to perform an average of three measurements while it seems necessary to make at least five measurements in patients with atrial fibrillation (59), an increase in VTI greater than or equal to 15% is considered a positive response to the filling. This parameter seems to be particularly reliable when performed by the same operator (23,24,60-63).
This value is considered equally reliable if the increase is greater than 10% as regards passive leg raising and mini fluid challenge (100 mL) (24,62,64). This measure is considered fast and easy to obtain and in accordance with numerous studies it is considered accurate and sensitive in predicting fluid responsiveness (65-70).
Caval index
The ultrasound study of inferior vena cava diameters (IVC) is now considered among the basic examinations in intensive care. One of the possible evaluations we can obtain is the CI, which represents the percentage of the change in vessel diameters during a respiratory cycle (1). These parameters are normally measured with transthoracic echocardiography, the vessel can be studied in a long-axis subcostal longitudinal view in M-mode, about 2 cm apart from right atrium junction and upstream to supra-hepatic vein (37,71,72). Fluid responsiveness can be considered when the CI reaches 12–18% variation in patients subjected to mechanical ventilation and 50% in patients with spontaneous breathing (1,23,72,73). This parameter as others already described previously (PPV and SVV) has some limits in its application due to the complexity of the systems it examines: heart-lung interactions (37,74). The most important limitations are patients not fully adapted to mechanical ventilation and those with increased intra-abdominal pressure, on the other hand this parameter is reliable in patients with cardiac arrhythmias (37).
Carotid Doppler measurements
The ultrasonographic study of the Doppler pulsed wave can be used to obtain two parameters: the carotid flow time (FTc) and the velocity time integral (VTI). These parameters come from similar studies performed on the aorta with the use of the transesophageal Doppler techniques (3,64,75) but they are easier to achieve and therefore more used. Velocity time integral (VTI) measured the blood flow through the carotid artery during systole. If we multiplied this parameter for the cross-sectional area of the carotid artery, this value asses the fraction of the stroke volume received by this vessel. FTc measures the amount of time spent in systole per cardiac cycle. A study by Marik et al. showed the correlation between two parameters: the SVV and the VTI changes, following the execution of the passive leg raise test with interesting results (r=0.59, P=0.0003) with a sensitivity of 94% and specificity of 86% (1,76). Jalil et al. compared the response to PLR test in both FTc and SVV changes and they observed a prediction in fluid responsiveness with AUROC of 0.75 with a sensitivity of 60% and specificity of 92% (77,78). These parameters can therefore be used as substitutes to SVV or cardiac output when this is impossible to perform due to the lack of suitable equipment and high costs of this technique.
End tidal CO2 variation
The end-tidal carbon dioxide (ETCO2) is a measure of the partial pressure of CO2 at the end of expiration and can be measured with a capnograph. The values of this parameter are determined by changes in cardiac output, therefore, it can be used as its indirect and non-invasive measure. The different values of ETCO2 registered previously and after a bolus of crystalloid or a simulated volume loading, is termed ΔETCO2 (1). Toupin et al. in their study they compare two parameters: ETCO2 and cardiac output obtained with the thermodilution technique, before, during and after having performed the passive leg raise test (PLR) (1,78). Patients were considered as fluid responders when an increase in ETCO2 ≥2 mmHg values was observed during the test with an odds ratio 7.3; 95% CI: 2.7–20.2; P<0.01; sensitivity 75%) and a negative predictive value of 86%.
A recent work Monnet et al. confront the values of ETCO2 and the cardiac index obtained with the PiCCO device, the test was performed on 40 mechanically ventilated patients who underwent PLR tests (1,79). In their publication a 5% increase in ETCO2 was associated with an increase in cardiac index of 15% after volume loading with a sensitivity of 71% (95% CI: 48–89%) and specificity of 100% (95% CI: 82–100%). this test therefore seems promising and easy to perform on patients in intensive care units and in the operating rooms (1).
Passive leg raising test (PLR)
Traditionally, passive lifting of the lower limbs has been a first aid maneuver practiced in patients with hypotension and/or cardiovascular shock. This maneuver causes a blood movement from the lower part of the body to thoracic area due to gravitational force, that increase the preload of cardiac chambers (80). It represents a preload test which consists in about 300 mL of blood (81), repeatable and it does not require infusion of fluids or colloids. Numerous studies have analyzed and confirmed the reliability of this maneuver and several meta-analyzes have been published (82,83). Monnet et al. (83) reported through a metanalysis in almost 1,000 adult patients and 21 studies included a pooled sensitivity of 85% and pooled sensibility of 91% of PLR test; the best sensitivity and specificity was found when the maneuver increased cardiac output above an average threshold of 10%. The great reliability of PLR test has allowed its inclusion in the Surviving Sepsis Campaign recommendation (84) and in the European Society of Intensive Care Medicine consensus conference (85). As recently reviewed (86), several aspects about the technique of performing the PLR must be analyzed. The reliability of PLR test cannot be based only in changes of arterial blood pressure and although a very good specificity, the sensitivity remains poor (82,83). Therefore, direct, continuously and real-time measurement of cardiac output is needed. The use of thermodilution in measuring cardiac output may not be sufficient because it is not done continuously. One of the characteristics of the studies in this field is that they have used non-invasive methods to establish the response to the PRL test (82,83). Among the first techniques used we find the esophageal Doppler, which studied changes in the aorta blood flow after the test was performed (87). Recently among the techniques best described in the studies, we find the velocity time integral of the left ventricular outflow tract obtained with echocardiography e measured before and after the PLR test. The modification of cardiac output in response to this test was also evaluated using peak velocity of carotid (76) and femoral (88) arteries and they seem to be good indicators. The argument that still has a conflict of results is bioreactance (76,89). In the ICU and operating rooms, non-invasive techniques such as pulse contour analysis of the arterial curve analyzed with photoplethysmography are in great demand, although for now the evidence on the reliability of this parameter during the test is weak. Another interesting non-invasive method to measure the response to PRL is the measurement of ETCO2 (90) variations. However, this method requires stable mechanical ventilation free from artifacts induced by the patient’s trigger. Passive lifting of the legs can be considered a safe test for preloading as the effects are completely reversible after the lower limbs return to the supine position (87,91), preventing the risk of overloading fluid and pulmonary edema. Moreover, this technique can mobilize not just the blood of lower limbs, but also blood of splanchnic district, increasing the sensitivity of the test but on the other hand the risk of false negative (92). The main advantages of this maneuver are the possibility of applying it to most critically ill patients, even in spontaneous breathing, low tidal volume, low lung compliance and with arrhythmias (55). However, there are some important limitations. The maneuver is not applicable in the absence of motorized beds capable of lifting the legs, in traumatized patients, in those with prone position and in patients in the operating room.
Fluid challenge
The fluid challenge is represented by the administration of an endovenous bolus of fluids (crystalloids or colloids) in a small and predetermined time interval for the purpose of measuring the subsequent hemodynamic response to its administration (93,94) The hemodynamic response to the fluid challenge cannot be reliable measured by monitoring only systemic arterial pressure (95,96) and 22% of false negative has been reported using this method alone (95). Therefore, it is now recommended to assess cardiac output and estimate the stroke volume instead of assessing the fluid-induced changes in central venous pressure (CVP) or pulmonary artery occlusion pressure (PAOP) (97). End-tidal carbon dioxide (ETCO2) is an alternative and non-invasive surrogate of cardiac output (98), maximal change in cardiac output should be assessed about 1 minute after ending fluid infusion (99). Since its administration is irreversible, classical fluid challenge can bring to fluid overload, particularly if repeated several times a day. To avoid this problem, a “mini” fluid challenge has been proposed for the first time by Muller et al. (48) giving 100 mL of colloid over 1 minute. The cardiac output was assessed measuring the outflow tract velocity time integral of left ventricle (measured with echocardiography), before and following the colloid administration. An increase with a threshold above 10% was more like to predict fluid responsiveness with good accuracy. In mechanically ventilated patients without arrhythmias, this method has great accuracy (area under the ROC curve =0.92, 95% CI: 0.78–0.98, r=0.81, P=0.0001). Other studies used pulse contour-analysis derived cardiac output (100), or changes in SVV (101) to reliably predict fluid responsiveness.
Other authors try to determine how small the bolus of fluids must be to guarantee a reliable fluid challenge by investigating the effects of different doses of intravenous fluids on changes in cardiac output, measured by pulse contour analysis and mean circulating filling pressure. Kim et al. (102) state that a bolus of 4 mL/kg over 5 min was the smallest volume that could reliably increase the mean circulating filling pressure and make fluid challenge interpretable in every circumstance. In conclusion, it can be stated that main limitation of a mini fluid challenge is that the small volume of fluid determines very small variations in cardiac output that require a very precise measurement technique.
End-expiratory occlusion test
The end-expiratory occlusion (EEO) test was developed by Monnet et al. and is performed interrupting mechanical ventilation for 15 seconds at the end of expiration and observing changes in the cardiac output. An increase in arterial pulse pressure or in pulse contour-derived cardiac index by more than 5% make this test positive and predicts a hemodynamic response to a fluid challenge with good accuracy (103). These findings have been confirmed also with a 30-seconds EEO test (104). The premise is that when EEO is performed, the cyclic impediment in venous return caused by each insufflation is interrupted and a duration of interruption of at least 15 seconds is sufficient to the bring the resulting increase in right ventricular stroke volume to cross the pulmonary circulation and increase also the left cardiac preload. The main advantages of this test are the ease of execution and the possibility to be performed in patients with acute respiratory distress syndrome (ARDS), a situation in which PPV and SVV are not reliable because a “lung-protective” ventilation with low tidal-volume and low lung compliance is applied (55,105). Silva et al. stated that predictive accuracy of the test is not altered in patients with ARDS and a level of positive end-expiratory pressure (PEEP) varying from 5 to 15 cmH2O (106). However, EOC test cannot be used in patients with spontaneous breathing on the ventilator that do not allow an expiratory pause of 15 seconds and, obviously, in patients who are not intubated. Furthermore, due to the short duration of the maneuver it is always necessary to have a precise and accurate monitoring of the hemodynamic response in real-time enough to detect a 5% change in the cardiac output. Jozwiak et al. stated that the end-expiratory occlusion test could be used with non-invasive techniques as the echocardiography, showing that changes in velocity-time integral (VTI) measured by echocardiography allow the EEO test to be monitored reliably (107). The diagnostic threshold was an increase VTI of 4%, however the precision of echocardiography was insufficient. For this reason, they added a 15-seconds end-inspiratory occlusion (EIO) which lowered cardiac output more in preload-dependent patients and predicted fluid responsiveness with a reliability similar to the EEO, with a greater diagnostic threshold of 15% (107).
Conclusions
Considering the Frank–Starling curve, the response to volume infusion is better when the cardiac preload is low than when it is high, however numerous studies have demonstrated that none of the static measures of cardiac preload is able to predict fluid responsiveness accurately. Although static markers of preload cannot be used to predict fluid responsiveness, they can be used to confirm that the fluid administered has actually filled the heart chambers, as well as to monitor fluid administration in order to decide the goal-directed therapy algorithms. Furthermore, they are good markers of cardiac function and structure and determinants of the pressure gradient organ perfusion. Although static measures are important for historical reasons and are widely used in clinical practice studies show that dynamic measures represent progress in the care of critically ill patients. Regarding dynamic measures and maneuvers, they are in general more reliable in predicting fluid responsiveness, but often they can be applied on selected groups of patients for example those subjected to mechanical ventilation with high tidal, they are more difficult to apply and interpret in patients with spontaneous breathing. Among the various tests examined, the PLR seems the most promising since it is applicable in a wider variety of patients, it is easy to perform and has fewer execution risks.
Acknowledgments
Funding: None.
Footnote
Conflicts of Interest: All authors have completed the ICMJE uniform disclosure form (available at 10.21037/jeccm.2020.03.03). The authors have no conflicts of interest to declare.
Ethical Statement: The authors are accountable for all aspects of the work in ensuring that questions related to the accuracy or integrity of any part of the work are appropriately investigated and resolved.
Open Access Statement: This is an Open Access article distributed in accordance with the Creative Commons Attribution-NonCommercial-NoDerivs 4.0 International License (CC BY-NC-ND 4.0), which permits the non-commercial replication and distribution of the article with the strict proviso that no changes or edits are made and the original work is properly cited (including links to both the formal publication through the relevant DOI and the license). See: https://creativecommons.org/licenses/by-nc-nd/4.0/.
References
- Jalil BA, Cavallazzi R. Predicting fluid responsiveness: A review of literature and a guide for the clinician. Am J Emerg Med 2018;36:2093-102. [Crossref] [PubMed]
- Reddi BAJ, Carpenter RHS. Venous excess: a new approach to cardiovascular control and its teaching. J Appl Physiol 2005;98:356-64. [Crossref] [PubMed]
- Jain RK, Antonio BL, Bowton DL, et al. Variability in central venous pressure measurements and the potential impact on fluid management. Shock 2010;33:253-7. [Crossref] [PubMed]
- Marik PE, Cavallazzi R. Does the Central Venous Pressure Predict Fluid Responsiveness? An Updated Meta-Analysis and a Plea for Some Common Sense*. Crit Care Med 2013;41:1774-81. [Crossref] [PubMed]
- Shippy CR, Appel PL, Shoemaker WC. Reliability of clinical monitoring to assess blood volume in critically ill patients. Crit Care Med 1984;12:107-12. [Crossref] [PubMed]
- Evans HL, Cuschieri J, Moore EE, et al. Inflammation and the Host Response to Injury, a Large-Scale Collaborative Project: Patient-Oriented Research Core Standard Operating Procedures for Clinical Care IX. Definitions for Complications of Clinical Care of Critically Injured Patients. J Trauma 2009;67:384-8. [Crossref] [PubMed]
- Cecconi M, Hofer C, Teboul JL, et al. Fluid challenges in intensive care: the FENICE study. Intensive Care Med 2015;41:1529-37. [Crossref] [PubMed]
- Cannesson M, Pestel G, Ricks C, et al. Hemodynamic monitoring and management in patients undergoing high risk surgery: A survey among North American and European anesthesiologists. Crit Care 2011;15:R197. [Crossref] [PubMed]
- Kumar A, Anel R, Bunnell E, et al. Preload-independent mechanisms contribute to increased stroke volume following large volume saline infusion in normal volunteers: a prospective interventional study. Crit Care 2004;8:R128. [Crossref] [PubMed]
- Osman D, Ridel C, Ray P, et al. Cardiac filling pressures are not appropriate to predict hemodynamic response to volume challenge*. Crit Care Med 2007;35:64-8. [Crossref] [PubMed]
- Michard F, Teboul JL. Predicting Fluid Responsiveness in ICU Patients. Chest 2002;121:2000-8. [Crossref] [PubMed]
- Michard F, Alaya S, Zarka V, et al. Global End-Diastolic Volume as an Indicator of Cardiac Preload in Patients with Septic Shock. Chest 2003;124:1900-8. [Crossref] [PubMed]
- Endo T, Kushimoto S, Yamanouchi S, et al. Limitations of global end-diastolic volume index as a parameter of cardiac preload in the early phase of severe sepsis: A subgroup analysis of a multicenter, prospective observational study. J Intensive Care 2013;1:11. [Crossref] [PubMed]
- Wiwatworapan W, Ratanajaratroj N, Sookananchai B. Correlation between inferior vena cava diameter and central venous pressure in critically ill patients. J Med Assoc Thai 2012;95:320-4. [PubMed]
- Brennan JM, Blair JE, Goonewardena S, et al. Reappraisal of the Use of Inferior Vena Cava for Estimating Right Atrial Pressure. J Am Soc Echocardiogr 2007;20:857-61. [Crossref] [PubMed]
- Vieillard-Baron A, Evrard B, Repessé X, et al. Limited value of end-expiratory inferior vena cava diameter to predict fluid responsiveness impact of intra-abdominal pressure. Intensive Care Med 2018;44:197-203. [Crossref] [PubMed]
- Guerin L, Monnet X, Teboul JL. Monitoring volume and fluid responsiveness: From static to dynamic indicators. Best Pract Res Clin Anaesthesiol 2013;27:177-85. [Crossref] [PubMed]
- Tavernier B, Makhotine O, Lebuffe G, et al. Systolic Pressure Variation as a Guide to Fluid Therapy in Patients with Sepsis-induced Hypotension. Anesthesiology 1998;89:1313-21. [Crossref] [PubMed]
- Bentzer P, Griesdale DE, Boyd J, et al. Will this hemodynamically unstable patient respond to a bolus of intravenous fluids? JAMA 2016;316:1298-309. [Crossref] [PubMed]
- Marik PE, Monnet X, Teboul JL. Hemodynamic parameters to guide fluid therapy. Ann Intensive Care 2011;1:1. [Crossref] [PubMed]
- Kumar A, Anel R, Bunnell E, et al. Pulmonary artery occlusion pressure and central venous pressure fail to predict ventricular filling volume, cardiac performance, or the response to volume infusion in normal subjects. Crit Care Med 2004;32:691-9. [Crossref] [PubMed]
- Monnet X, Teboul JL. Invasive measures of left ventricular preload. Curr Opin Crit Care 2006;12:235-40. [Crossref] [PubMed]
- Feissel M, Michard F, Faller JP, et al. The respiratory variation in inferior vena cava diameter as a guide to fluid therapy. Intensive Care Med 2004;30:1834-7. [Crossref] [PubMed]
- Lamia B, Ochagavia A, Monnet X, et al. Echocardiographic prediction of volume responsiveness in critically ill patients with spontaneously breathing activity. Intensive Care Med 2007;33:1125-32. [Crossref] [PubMed]
- Mekontso-Dessap A, Tual L, Kirsch M, et al. B-type natriuretic peptide to assess haemodynamic status after cardiac surgery. Br J Anaesth 2006;97:777-82. [Crossref] [PubMed]
- Chung CS, Shmuylovich L, Kovács SJ. What global diastolic function is, what it is not, and how to measure it. Am J Physiol Heart Circ Physiol 2015;309:H1392-406. [Crossref] [PubMed]
- Garcia MJ, Thomas JD, Klein AL. New Doppler echocardiographic applications for the study of diastolic function. J Am Coll Cardiol 1998;32:865-75. [Crossref] [PubMed]
- Jacques DC, Pinsky MR, Severyn D, et al. Influence of alterations in loading on mitral annular velocity by tissue doppler echocardiography and its associated ability to predict filling pressures. Chest 2004;126:1910-8. [Crossref] [PubMed]
- Nagueh SF, Smiseth OA, Appleton CP, et al. Recommendations for the Evaluation of Left Ventricular Diastolic Function by Echocardiography: An Update from the American Society of Echocardiography and the European Association of Cardiovascular Imaging. J Am Soc Echocardiogr 2016;29:277-314. [Crossref] [PubMed]
- Pirracchio R, Cholley B, Hert S, De , et al. Diastolic heart failure in anaesthesia and critical care. Br J Anaesth 2007;98:707-21. [Crossref] [PubMed]
- Nagueh SF, Middleton KJ, Kopelen HA, et al. Doppler tissue imaging: A noninvasive technique for evaluation of left ventricular relaxation and estimation of filling pressures. J Am Coll Cardiol 1997;30:1527-33. [Crossref] [PubMed]
- Vignon P, Allot V, Lesage J, et al. Diagnosis of left ventricular diastolic dysfunction in the setting of acute changes in loading conditions. Crit Care 2007;11:R43. [Crossref] [PubMed]
- Dhainaut JF, Huyghebaert MF, Monsallier JF, et al. Coronary hemodynamics and myocardial metabolism of lactate, free fatty acids, glucose, and ketones in patients with septic shock. Circulation 1987;75:533-41. [Crossref] [PubMed]
- Sanfilippo F, Corredor C, Arcadipane A, et al. Tissue Doppler assessment of diastolic function and relationship with mortality in critically ill septic patients: a systematic review and meta-analysis. Br J Anaesth 2017;119:583-94. [Crossref] [PubMed]
- Mahjoub Y, Benoit-Fallet H, Airapetian N, et al. Improvement of left ventricular relaxation as assessed by tissue Doppler imaging in fluid-responsive critically ill septic patients. Intensive Care Med 2012;38:1461-70. [Crossref] [PubMed]
- Vieillard-Baron A, Cecconi M. Understanding cardiac failure in sepsis. Intensive Care Med 2014;40:1560-3. [Crossref] [PubMed]
- Jozwiak M, Monnet X, Teboul JL. Prediction of fluid responsiveness in ventilated patients. Ann Transl Med 2018;6:352. [Crossref] [PubMed]
- Wong FWH. Pulsus paradoxus in ventilated and non-ventilated patients. Dynamics 2007;18:16-8. [PubMed]
- Michard F, Teboul JL. Using heart-lung interactions to assess fluid responsiveness during mechanical ventilation. Crit Care 2000;4:282-9. [Crossref] [PubMed]
- Monnet X, Marik PE, Teboul JL. Prediction of fluid responsiveness: an update. Ann Intensive Care 2016;6:111. [Crossref] [PubMed]
- Myatra SN, Prabu NR, Divatia JV, et al. The Changes in Pulse Pressure Variation or Stroke Volume Variation After a “Tidal Volume Challenge” Reliably Predict Fluid Responsiveness During Low Tidal Volume Ventilation*. Crit Care Med 2017;45:415-21. [Crossref] [PubMed]
- Berkenstadt H, Margalit N, Hadani M, et al. Stroke volume variation as a predictor of fluid responsiveness in patients undergoing brain surgery. Anesth Analg 2001;92:984-9. [Crossref] [PubMed]
- Piccioni F, Bernasconi F, Tramontano GTA, et al. A systematic review of pulse pressure variation and stroke volume variation to predict fluid responsiveness during cardiac and thoracic surgery. J Clin Monit Comput 2017;31:677-84. [Crossref] [PubMed]
- Reuter DA, Kirchner A, Felbinger TW, et al. Usefulness of left ventricular stroke volume variation to assess fluid responsiveness in patients with reduced cardiac function. Crit Care Med 2003;31:1399-404. [Crossref] [PubMed]
- Zhang Z, Lu B, Sheng X, et al. Accuracy of stroke volume variation in predicting fluid responsiveness: a systematic review and meta-analysis. J Anesth 2011;25:904-16. [Crossref] [PubMed]
- Teboul JL, Monnet X. Prediction of volume responsiveness in critically ill patients with spontaneous breathing activity. Curr Opin Crit Care 2008;14:334-9. [Crossref] [PubMed]
- De Backer D, Heenen S, Piagnerelli M, et al. Pulse pressure variations to predict fluid responsiveness: Influence of tidal volume. Intensive Care Med 2005;31:517-23. [Crossref] [PubMed]
- Muller L, Louart G, Bousquet PJ, et al. The influence of the airway driving pressure on pulsed pressure variation as a predictor of fluid responsiveness. Intensive Care Med 2010;36:496-503. [Crossref] [PubMed]
- Mahjoub Y, Lejeune V, Muller L, et al. Evaluation of pulse pressure variation validity criteria in critically ill patients: A prospective observational multicentre point-prevalence study. Br J Anaesth 2014;112:681-5. [Crossref] [PubMed]
- Michard F. Changes in arterial pressure during mechanical ventilation. Anesthesiology 2005;103:419-28. [Crossref] [PubMed]
- Michard F, Boussat S, Chemla D, et al. Relation between respiratory changes in arterial pulse pressure and fluid responsiveness in septic patients with acute circulatory failure. Am J Respir Crit Care Med 2000;162:134-8. [Crossref] [PubMed]
- Marik PE, Cavallazzi R, Vasu T, et al. Dynamic changes in arterial waveform derived variables and fluid responsiveness in mechanically ventilated patients: A systematic review of the literature*. Crit Care Med 2009;37:2642-7. [Crossref] [PubMed]
- Yang X, Du B. Does pulse pressure variation predict fluid responsiveness in critically ill patients? A systematic review and meta-analysis. Crit Care 2014;18:650. [Crossref] [PubMed]
- Michard F, Chemla D, Teboul JL. Applicability of pulse pressure variation: How many shades of grey? Crit Care 2015;19:144. [Crossref] [PubMed]
- Monnet X, Bleibtreu A, Ferré A, et al. Passive leg-raising and end-expiratory occlusion tests perform better than pulse pressure variation in patients with low respiratory system compliance*. Crit Care Med 2012;40:152-7. [Crossref] [PubMed]
- Cannesson M, Le Manach Y, Hofer CK, et al. Assessing the diagnostic accuracy of pulse pressure variations for the prediction of fluid responsiveness: A “gray zone” approach. Anesthesiology 2011;115:231-41. [Crossref] [PubMed]
- Carlos RV, Bittar CS, Lopes MR, et al. Systolic pressure variation as diagnostic method for hypovolemia during anesthesia for cardiac surgery. Rev Bras Anestesiol 2005;55:3-18. [Crossref] [PubMed]
- Jozwiak M, Mercado P, Teboul JL, et al. What is the lowest change in cardiac output that transthoracic echocardiography can detect? Crit Care 2019;23:116. [Crossref] [PubMed]
- Lang RM, Badano LP, Victor MA, et al. Recommendations for cardiac chamber quantification by echocardiography in adults: An update from the American Society of Echocardiography and the European Association of Cardiovascular Imaging. J Am Soc Echocardiogr 2015;28:1-39.e14. [Crossref] [PubMed]
- Feissel M, Michard F, Mangin I, et al. Respiratory changes in aortic blood velocity as an indicator of fluid responsiveness in ventilated patients with septic shock. Chest 2001;119:867-73. [Crossref] [PubMed]
- Muller L, Toumi M, Bousquet PJ, et al. An increase in aortic blood flow after an infusion of 100 ml colloid over 1 minute can predict fluid responsiveness: The mini-fluid challenge study. Anesthesiology 2011;115:541-7. [Crossref] [PubMed]
- Guinot PG, Zogheib E, Detave M, et al. Passive leg raising can predict fluid responsiveness in patients placed on venovenous extracorporeal membrane oxygenation. Crit Care 2011;15:R216. [Crossref] [PubMed]
- Charbonneau H, Riu B, Faron M, et al. Predicting preload responsiveness using simultaneous recordings of inferior and superior vena cavae diameters. Crit Care 2014;18:473. [Crossref] [PubMed]
- Muller L, Toumi M, Bousquet PJ, et al. An Increase in Aortic Blood Flow after an Infusion of 100 ml Colloid over 1 Minute Can Predict Fluid Responsiveness. Anesthesiology 2011;115:541-7. [Crossref] [PubMed]
- Wu Y, Zhou S, Zhou Z, et al. A 10-second fluid challenge guided by transthoracic echocardiography can predict fluid responsiveness. Crit Care 2014;18:R108. [Crossref] [PubMed]
- Brun C, Zieleskiewicz L, Textoris J, et al. Prediction of fluid responsiveness in severe preeclamptic patients with oliguria. Intensive Care Med 2013;39:593-600. [Crossref] [PubMed]
- Lukito V, Djer MM, Pudjiadi AH, et al. The role of passive leg raising to predict fluid responsiveness in pediatric intensive care unit patients. Pediatr Crit Care Med 2012;13:e155-60. [Crossref] [PubMed]
- Broch O, Renner J, Gruenewald M, et al. Variation of left ventricular outflow tract velocity and global end-diastolic volume index reliably predict fluid responsiveness in cardiac surgery patients. J Crit Care 2012;27:325.e7-13. [Crossref] [PubMed]
- Feissel M, Teboul JL, Merlani P, et al. Plethysmographic dynamic indices predict fluid responsiveness in septic ventilated patients. Intensive Care Med 2007;33:993-9. [Crossref] [PubMed]
- Slama M, Masson H, Teboul JL, et al. Respiratory variations of aortic VTI: A new index of hypovolemia and fluid responsiveness. Am J Physiol Heart Circ Physiol 2002;283:H1729-33. [Crossref] [PubMed]
- Wallace DJ, Allison M, Stone MB. Inferior Vena Cava Percentage Collapse During Respiration Is Affected by the Sampling Location: An Ultrasound Study in Healthy Volunteers. Acad Emerg Med 2010;17:96-9. [Crossref] [PubMed]
- Airapetian N, Maizel J, Alyamani O, et al. Does inferior vena cava respiratory variability predict fluid responsiveness in spontaneously breathing patients? Crit Care 2015;19:400. [Crossref] [PubMed]
- Barbier C, Loubières Y, Schmit C, et al. Respiratory changes in inferior vena cava diameter are helpful in predicting fluid responsiveness in ventilated septic patients. Intensive Care Med 2004;30:1740-6. [Crossref] [PubMed]
- Via G, Tavazzi G, Price S. Ten situations where inferior vena cava ultrasound may fail to accurately predict fluid responsiveness: a physiologically based point of view. Intensive Care Med 2016;42:1164-7. [Crossref] [PubMed]
- Lafanechère A, Pène F, Goulenok C, et al. Changes in aortic blood flow induced by passive leg raising predict fluid responsiveness in critically ill patients. Crit Care 2006;10:R132. [Crossref] [PubMed]
- Marik PE, Levitov A, Young A, et al. The Use of Bioreactance and Carotid Doppler to Determine Volume Responsiveness and Blood Flow Redistribution Following Passive Leg Raising in Hemodynamically Unstable Patients. Chest 2013;143:364-70. [Crossref] [PubMed]
- Jalil B, Thompson P, Cavallazzi R, et al. Comparing Changes in Carotid Flow Time and Stroke Volume Induced by Passive Leg Raising. Am J Med Sci 2018;355:168-73. [Crossref] [PubMed]
- Toupin F, Clairoux A, Deschamps A, et al. Assessment of fluid responsiveness with end-tidal carbon dioxide using a simplified passive leg raising maneuver: a prospective observational study. Can J Anesth Can d’anesthésie 2016;63:1033-41.
- Monnet X, Bataille A, Magalhaes E, et al. End-tidal carbon dioxide is better than arterial pressure for predicting volume responsiveness by the passive leg raising test. Intensive Care Med 2013;39:93-100. [Crossref] [PubMed]
- Boulain T, Achard JM, Teboul JL, et al. Changes in BP induced by passive leg raising predict response to fluid loading in critically ill patients. Chest 2002;121:1245-52. [Crossref] [PubMed]
- Jabot J, Teboul JL, Richard C, et al. Passive leg raising for predicting fluid responsiveness: Importance of the postural change. Intensive Care Med 2009;35:85-90. [Crossref] [PubMed]
- Cherpanath TG V., Hirsch A, Geerts BF, et al. Predicting Fluid Responsiveness by Passive Leg Raising. Crit Care Med 2016;44:981-91. [Crossref] [PubMed]
- Monnet X, Marik P, Teboul JL. Passive leg raising for predicting fluid responsiveness: a systematic review and meta-analysis. Intensive Care Med 2016;42:1935-47. [Crossref] [PubMed]
- Levy MM, Evans LE, Rhodes A. The Surviving Sepsis Campaign Bundle: 2018 update. Intensive Care Med 2018;44:925-8. [Crossref] [PubMed]
- Cecconi M, Backer D, De , Antonelli M, et al. Consensus on circulatory shock and hemodynamic monitoring. Task force of the European Society of Intensive Care Medicine. Intensive Care Med 2014;40:1795-815. [Crossref] [PubMed]
- Monnet X, Teboul JL. Passive leg raising: five rules, not a drop of fluid! Crit Care 2015;19:18. [Crossref] [PubMed]
- Monnet X, Rienzo M, Osman D, et al. Passive leg raising predicts fluid responsiveness in the critically ill*. Crit Care Med 2006;34:1402-7. [Crossref] [PubMed]
- Préau S, Saulnier F, Dewavrin F, et al. Passive leg raising is predictive of fluid responsiveness in spontaneously breathing patients with severe sepsis or acute pancreatitis*. Crit Care Med 2010;38:819-25. [Crossref] [PubMed]
- Kupersztych-Hagege E, Teboul JL, Artigas A, et al. Bioreactance is not reliable for estimating cardiac output and the effects of passive leg raising in critically ill patients. Br J Anaesth 2013;111:961-6. [Crossref] [PubMed]
- Xiao-ting W, Hua Z, Da-wei L, et al. Changes in end-tidal CO2 could predict fluid responsiveness in the passive leg raising test but not in the mini-fluid challenge test: A prospective and observational study. J Crit Care 2015;30:1061-6. [Crossref] [PubMed]
- Monnet X, Teboul J-L. Passive leg raising. Intensive Care Med 2008;34:659-63. [Crossref] [PubMed]
- Cavallaro F, Sandroni C, Marano C, et al. Diagnostic accuracy of passive leg raising for prediction of fluid responsiveness in adults: systematic review and meta-analysis of clinical studies. Intensive Care Med 2010;36:1475-83. [Crossref] [PubMed]
- Vincent JL, Weil MH. Fluid challenge revisited. Crit Care Med 2006;34:1333-7. [Crossref] [PubMed]
- Weil MH, Henning RJ. New concepts in the diagnosis and fluid treatment of circulatory shock. Thirteenth annual Becton, Dickinson and Company Oscar Schwidetsky Memorial Lecture. Anesth Analg 1979;58:124-32. [Crossref] [PubMed]
- Monnet X, Letierce A, Hamzaoui O, et al. Arterial pressure allows monitoring the changes in cardiac output induced by volume expansion but not by norepinephrine. Crit Care Med 2011;39:1394-9. [Crossref] [PubMed]
- Pierrakos C, Velissaris D, Scolletta S, et al. Can changes in arterial pressure be used to detect changes in cardiac index during fluid challenge in patients with septic shock? Intensive Care Med 2012;38:422-8. [Crossref] [PubMed]
- Rhodes A, Evans LE, Alhazzani W, et al. Surviving Sepsis Campaign: International Guidelines for Management of Sepsis and Septic Shock: 2016. Intensive Care Med 2017;43:304-77. [Crossref] [PubMed]
- Lakhal K, Nay MA, Kamel T, et al. Change in end-tidal carbon dioxide outperforms other surrogates for change in cardiac output during fluid challenge. Br J Anaesth 2017;118:355-62. [Crossref] [PubMed]
- Aya HD, Ster IC, Fletcher N, et al. Pharmacodynamic analysis of a fluid challenge. Crit Care Med 2016;44:880-91. [Crossref] [PubMed]
- Biais M, Courson H, De , Lanchon R, et al. Mini-fluid Challenge of 100 ml of Crystalloid Predicts Fluid Responsiveness in the Operating Room. Anesthesiology 2017;127:450-6. [Crossref] [PubMed]
- Mallat J, Meddour M, Durville E, et al. Decrease in pulse pressure and stroke volume variations after mini-fluid challenge accurately predicts fluid responsiveness. Br J Anaesth 2015;115:449-56. [Crossref] [PubMed]
- Kim KM, Gwak MS, Choi SJ, et al. Pulse pressure variation and stroke volume variation to predict fluid responsiveness in patients undergoing carotid endarterectomy. Korean J Anesthesiol 2013;65:237-43. [Crossref] [PubMed]
- Monnet X, Osman D, Ridel C, et al. Predicting volume responsiveness by using the end-expiratory occlusion in mechanically ventilated intensive care unit patients. Crit Care Med 2009;37:951-6. [Crossref] [PubMed]
- Biais M, Larghi M, Henriot J, et al. End-expiratory occlusion test predicts fluid responsiveness in patients with protective ventilation in the operating room. Anesth Analg 2017;125:1889-95. [Crossref] [PubMed]
- Teboul JL, Monnet X. Pulse pressure variation and ARDS. Minerva Anestesiol 2013;79:398-407. [PubMed]
- Silva S, Jozwiak M, Teboul JL, et al. End-Expiratory Occlusion Test Predicts Preload Responsiveness Independently of Positive End-Expiratory Pressure During Acute Respiratory Distress Syndrome. Crit Care Med 2013;41:1692-701. [Crossref] [PubMed]
- Jozwiak M, Depret F, Teboul JL, et al. Predicting Fluid Responsiveness in Critically Ill Patients by Using Combined End-Expiratory and End-Inspiratory Occlusions With Echocardiography. Crit Care Med 2017;45:e1131-8. [Crossref] [PubMed]
Cite this article as: Musu M, Guddelmoni L, Murgia F, Mura S, Bonu F, Mura P, Finco G. Prediction of fluid responsiveness in ventilated critically ill patients. J Emerg Crit Care Med 2020;4:26.